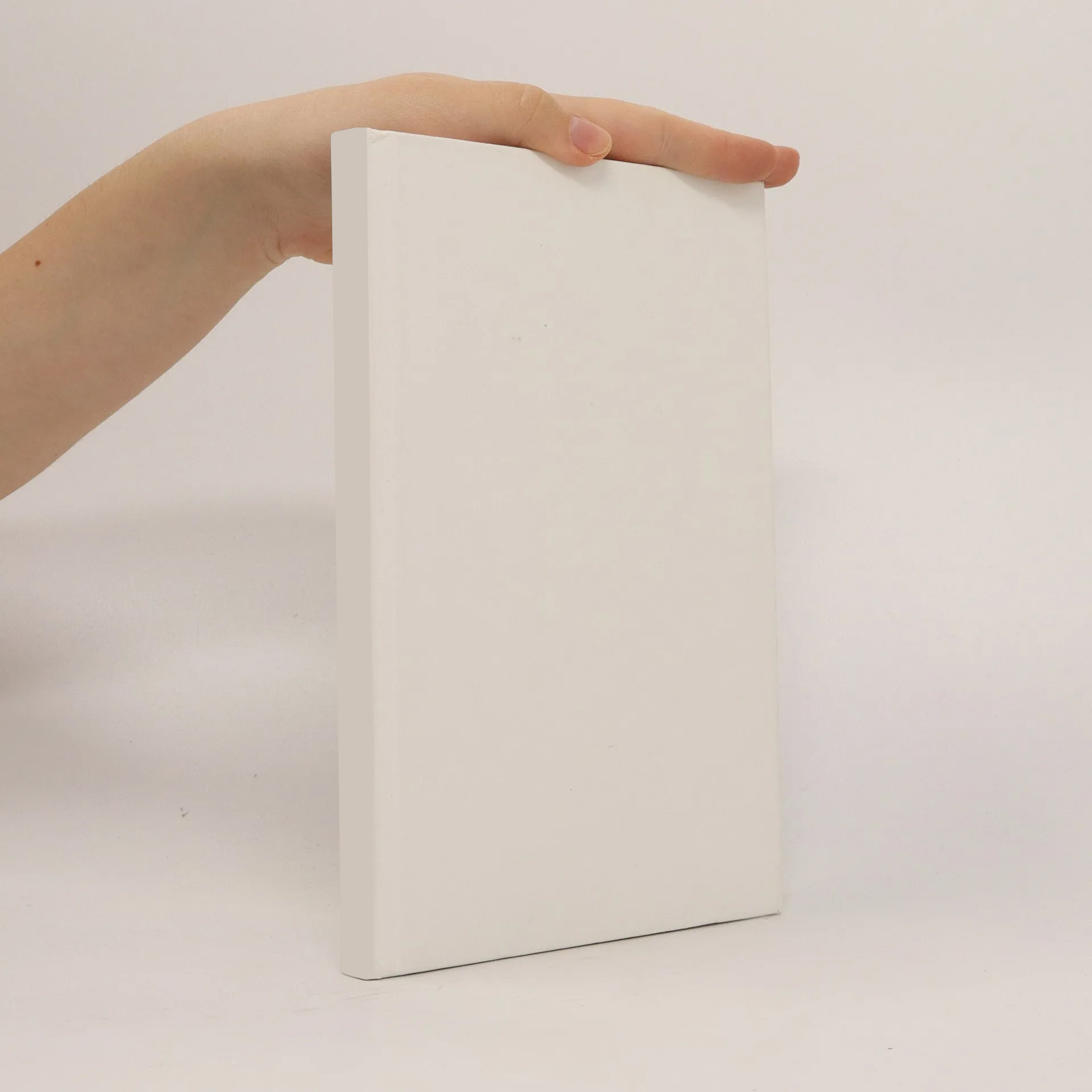
Parameter
Mehr zum Buch
Over the last centuries, it has been realized that atoms and molecules are the building blocks of life and the world surrounding us. This awareness led to the development of molecular physics as one of the key research fields to investigate e. g. chemical interactions, the role of molecules in biological systems, and large scale astrophysical processes in the universe. To understand these often extremely complex mechanisms, it is crucial to have a complete picture of the inherent properties and interactions of the atoms and molecules involved. These properties can be precisely studied using gas-phase samples, since any interactions between the particles and their environment are then absent. Room-temperature molecules and atoms in the gas-phase, however, move around with very high velocities on the order of a few 100 ms−1. In a very simple picture, one can argue that if an object moves very fast, its details are not accessible and thus remain hidden. Likewise, objects that are slowed down can be studied in much higher detail. The equivalent to slowing down molecules and atoms is to cool them to a lower temperature. To translate this into physics terms, a higher measurement sensitivity can be achieved by increasing the interaction time of the particles with the probing system, for instance, with a laser beam. The quality of a measurement can be even further increased by confining particles in a trapping field after cooling, allowing for the longest possible measurement times. Since the development of laser cooling in the 1970s, the field of atomic and molecular physics has experienced a remarkable growth. Over the years, mature methods to manipulate the motional degrees of freedom have been developed which led to a large new field of research studying the rich physics of cold particle interactions. Among these phenomena, Bose-Einstein-Condensation (BEC) can be regarded as one of the milestones of this new field. Such a BEC can be formed when the wave functions of single particles start to overlap at decreasing temperatures and increasing phase-space densities. As a result, macroscopic signatures of quantum phenomena become visible, an effect that was first observed in 1995 by three independent research groups [1, 2, 3], and has been rewarded with the Nobel price in physics in 2001. Soon after this, further breakthroughs have been reached like the achievement of Fermi degeneracy in atomic vapors [4] or the realization of atom lasers [5,6,7,8]. For a long time, most of the work in this field was restricted to atoms only, since a general and efficient cooling mechanism, equivalent to laser cooling, was lacking for molecules. Since molecules have a more complex inner structure, closed transitions - a premise for laser cooling - are hard to find. However, it is exactly this complexity of molecules that offers the perspectives to study new and exciting effects that are much harder to address or are even absent in atoms [9, 10, 11]. As a consequence, studies of cold molecules have moved more and more into the focus of modern research, a trend which also led to the development of suitable cooling mechanisms. Some of the prospects that cold molecules offer shall be briefly depicted here.
Buchkauf
Buffer gas cooling and magnetic trapping of CrH and MnH molecules, Michael Stoll
- Sprache
- Erscheinungsdatum
- 2008
Lieferung
Zahlungsmethoden
Keiner hat bisher bewertet.